GaN, GaAs, Or SiGe – Which Is The Best Semiconductor Material?

By John Oncea, Editor
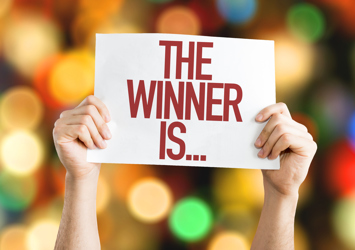
GaN, GaAs, and SiGe offer unique properties for various semiconductor applications. Which is best? The answer might surprise you.
What comes to mind when you think of “the best?” This is a trick question of sorts as the only correct answer is Tina Turner. *
But this is RF Globalnet, not Rolling Stone. So let’s rethink our answer in terms of semiconductor materials. Which is the best: GaN, GaAs, or SiGe? The answer to that is a bit more complicated but far more appropriate for our audience.
Anybody working with semiconductor chips realizes, given their importance in electrical devices, that “it is impossible to ignore the materials that they are made of,” writes ICRFQ. “After all, the material will determine several aspects of the final product. For example, do you know the material of the chip will affect its reliability?”
So, before determining the best material, let’s define what gallium nitride (GaN), gallium arsenide (GaAs), and silicon germanium (SiGe) are.
* Imagine my surprise when researching this article and discovering you can buy tickets to see Tina Turner – famously dead since May 2023 – performing live! Oh, wait. Those tickets are for Tina – The Tina Turner Musical, currently rolling across America and, “Garnering rave reviews from critics and the public alike.” **
** Also, Bonnie Tyler – still alive and touring Europe – was the first person to record “The Best,” more than a year and a half before Turner’s cover. Oh, and the sax solo on Tuner’s cover? It was performed by Edgar Winter!
GaN: Better Be Good To Me
Gallium nitride (GaN) is gaining in popularity as a semiconductor material because it is possible to fabricate many devices with performance that cannot be equaled by silicon, writes Electronics Notes. GaN technology – known for being a key component of Blu-ray discs – “is also being increasingly used in semiconductor power devices, RF, and microwave semiconductor components, as well as lasers and photonics. Devices including GaN FETs or GaN HEMTs are increasingly being seen for both RF design and general power electronics applications.”
GaN is created by combining gallium and nitrogen and is a wide bandgap semiconductor material with a hard, hexagonal crystal structure. “Bandgap is the energy needed to free an electron from its orbit around the nucleus and, at 3.4 eV, the bandgap of GaN is over three times that of silicon, thus the designation ‘wide’ bandgap or WBG,” Navitas writes.
The bandgap of a material determines the strength of the electric field it can handle. GaN has a wider bandgap, which allows the creation of semiconductors with very short or narrow depletion regions. This leads to device structures with high carrier density. With smaller transistors and shorter current paths, ultra-low resistance and capacitance are achieved, enabling speeds up to 100 times faster.
GaN technology is capable of handling larger electric fields in a much smaller size compared to conventional silicon. It also provides significantly faster switching. Moreover, GaN technologies can operate at higher maximum temperatures than their silicon-based counterparts.
Not for nothing, but in the U.S. and U.K., gallium nitride is GaN. But, according to Navitas, in China, this material is referred to as nitride of gallium or NGa. In addition, the Chinese version of the formula contains three symbols – one each for gallium (镓) and nitrogen (氮) and a 化 to connect them.
GaAs: It Takes Two
Gallium arsenide (GaAs) is a semiconductor material that excels in high-speed and high-frequency applications. It has higher electron mobility, a wider bandgap, and a higher breakdown voltage than silicon, making it suitable for high-power applications.
“GaAs tend to have low noise,” ICRGQ writes. “It has high immunity to electric noise that can interfere with the flow of electrons. Radiofrequency is the most common noise that affects most semiconductor chips. Minimum noise translates to high accuracy and better performance.”
GaAs “is a compound semiconductor consisting of gallium and arsenic atoms. It offers a direct bandgap, which is advantageous for optoelectronic devices like lasers and photodetectors.,” Wafer World writes. “GaAs wafers exhibit higher electron mobility and faster electron velocity, making them ideal for high-frequency and high-speed applications such as RF amplifiers and satellite communications. “
GaAs wafers play a crucial role in the production of various electronic devices. These wafers are made up of single crystals of gallium arsenide, which exhibit unique electrical and optical properties. Techniques such as molecular beam epitaxy (MBE) or metalorganic vapor phase epitaxy (MOVPE) are typically used to grow GaAs wafers.
“GaAs is a III-V compound semiconductor with a direct bandgap, allowing for efficient emission and absorption of light in the infrared and visible light spectrum,” adds Nanografi Nano Technology. “GaAs wafers have high electron mobility, surpassing silicon, making them suitable for high-speed applications such as microwave devices, high-speed transistors, and integrated circuits (ICs).”
SiGe: Let’s Stay Together
“Silicon germanium (SiGe) alloys have attracted a lot of interest in semiconductor research because of their special structure and beneficial physical characteristics,” AnySilicon writes. “SiGe alloys are preferable to their elemental equivalents because they can have adjustable properties due to their combination of germanium (Ge) and silicon (Si).”
SiGe technology is becoming increasingly popular in modern RF components. This technology allows for the creation of high-speed transistors, which enables the development of extremely fast communication systems. This effect has been particularly beneficial in the telecom sector, making advanced technologies like 5G networks more feasible. The integration of SiGe has improved signal processing capabilities and has been crucial in the development of wireless connectivity, satellite communication, and radar systems.
“SiGe has better electron mobility than either pure silicon or germanium, which allows for better electrical performance,” writes AnySilicon. “Higher carrier mobility is introduced by adding germanium to the silicon lattice structure, which is essential for the advancement of high-speed electronic devices. Additionally, the alloy’s tunable bandgap enables customized electrical characteristics to meet particular application needs.”
The use of SiGe technology in modern RF components is a remarkable trend. By leveraging SiGe's unique properties, high-speed transistors can be produced, which enables the development of lightning-fast communication systems. This impact is particularly noticeable in the telecom sector, where it makes 5G networks and other advanced technologies more viable. The incorporation of SiGe has enhanced signal processing capabilities and has been critical to the development of wireless connectivity, satellite communication, and radar systems.
Back Where You Started
Determining the best semiconductor material depends on many factors, including the specific application and requirements. Each material has its advantages and disadvantages:
GaN offers high electron mobility, allowing it to operate at high frequencies and high-power densities. It also exhibits excellent thermal stability and can handle high temperatures, making it suitable for power electronics, LEDs, and RF devices. However, GaN fabrication can be more challenging and expensive compared to other materials. Additionally, the availability of high-quality GaN substrates is limited.
GaAs has high electron mobility, making it suitable for high-frequency applications such as microwave devices, RF amplifiers, and solar cells. It also has good noise performance and is widely used in optoelectronic devices like LEDs and laser diodes. That said, GaAs can be more costly than silicon and may have lower breakdown voltage. It is also sensitive to temperature variations.
SiGe combines the benefits of silicon (low-cost, mature manufacturing processes) with the high carrier mobility of germanium, making it suitable for high-speed integrated circuits (ICs), especially in RF and mixed-signal applications. SiGe may have limitations in terms of maximum operating frequency compared to GaAs or GaN. It may also require more complex manufacturing processes than pure silicon.
When choosing the best material for a particular application, there are several factors to consider, such as cost, performance requirements, operating conditions, and available fabrication technologies. If power is your primary concern, GaN is the ideal choice as it can reach significant frequencies of up to 100GHz while maintaining high power levels. However, GaN is only suitable for backhaul and base-station-type applications.
If RF performance is the only criterion, then GaAs is the best option. It offers superior noise performance, super high ft (above 1THz), and moderate power levels. However, GaAs has lower yields, requires special packaging, and has a low integration density.
SiGe is a compromise material that most BiCMOS processes use. It provides a balance between decent RF performance (ft above 350 GHz), acceptable noise figure, and power output with higher integration density, cheaper and reliable CMOS production technology, and the availability of digital and analog functions.
The Best Is …
Cubic boron arsenide?
“Cubic boron arsenide was first synthesized just a few years ago by scientists working with Zhifeng Ren at the University of Houston,” AZoNano writes. “In 2015, Ren and his colleagues grew crystals of the material from boron and arsenic, later discovering its promising semiconductor properties in 2018. Boron arsenide (or BAs) has a lattice constant of 0.4777 nm and its indirect band gap is 1.82 eV. It melts at 2076 C, has an optical refractive index of 3.29 at 657 nm wavelength, and elastic modulus is 326 GPa.”
In 2022, Physics World recognized two experiments as “Top 10 Breakthroughs” of the year. These experiments showcased the superconductive potential of cubic boron arsenide which was largely due to the material’s ability to conduct both electrons and electron-holes extremely well. Unlike most other semiconductors, which only allow one type of electron particle to travel through them efficiently, this material’s efficiency is determined by the less conductive of the two.
“Cubic boron arsenide is also highly thermally conductive, theoretically reaching 2,200 W/(m-K),” writes AZoNano. “It was only around 190 W/(m-K) when it was first synthesized due to defects in the material; scientists improved the process to remove defects and have now created cubic boron arsenide with thermal conductivity of 1000 W/(m-K). This makes it comparable to extremely thermally conductive materials like diamond and graphite, and much more thermally conductive than other semiconductor materials.”
“The potential of this material is tremendous,” said Ren. “While work to consistently produce larger crystals with uniform properties is ongoing, the result could have an even bigger impact on the field than the silicon wafer.”
Boron arsenide is being researched for use in electronics thermal management due to its high electronic and thermal conductivity. Thermal management is crucial in modern electronic systems where heat energy needs to be dissipated across electronics such as computers and cars. Some researchers are exploring novel semiconductors to address this issue. Cubic boron arsenide and boron phosphide are potential replacements for silicon in chips and can serve as both cooling and electronically conductive substrates.