Semiconductors At A Crossroads: Silicon, GaN, Or Something Else?

By John Oncea, Editor
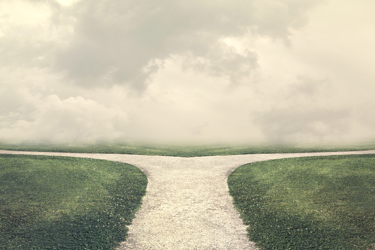
GaN is a semiconductor material with several advantages, including energy efficiency, smaller system sizes, and high-temperature operation. However, GaN faces challenges like manufacturing complexity and material defects. Will it replace silicon? Or is there a dark horse out there, waiting to knock them both off?
There is, I think it’s safe to assume, agreement on the pros and cons of Gallium Nitride (GaN), a binary III/V direct bandgap semiconductor well-suited for high-power transistors capable of operating at high temperatures, according to GaN Systems.
GaN – used in LEDs to emit blue light for disc-reading in Blu-ray, as semiconductor power devices, as power supplies and adaptors for PCs, portable electronics, and wall USB chargers, and more – brings with it several advantages, Gan Systems writes. These include:
- Reduced energy costs: Because GaN semiconductors are inherently more efficient than silicon, less energy is expended as heat, resulting in smaller system sizes and material costs.
- Higher power density (smaller volume): Higher switching frequencies and operational temperatures than silicon result in lower cooling requirements, smaller heat sinks, conversion from liquid cooling to air cooling, eliminating fans, and reduced magnetics.
- Higher switching frequency: The higher switching frequencies for GaN devices allow smaller inductors and capacitors to be used in power circuits. The inductance and capacitance scale down in proportion to the frequency – a 10X increase in frequency produces a 10X decrease in the capacitance and inductance. This can result in an enormous decrease in weight and volume, as well as cost. In addition, higher frequency can result in less acoustic noise in motor drive applications. High frequency also enables wireless power transfer at higher powers, more spatial freedom, and bigger transmit-to-receive air gaps.
- Lower system cost: While GaN semiconductors are generally higher cost than silicon, system-level cost reductions result through the use of GaN by reducing the size/costs of other components such as passive inductive and capacitive circuit elements, filters, cooling, etc. Savings range from 10-20%.
“A challenge currently present is the manufacturing complexity and cost associated with GaN-based devices, writes Cadence Design Systems. “The fabrication of GaN semiconductors requires specialized techniques and equipment, which can be more complex and expensive compared to silicon-based processes. The unique properties of GaN, such as its lattice mismatch with commonly used substrates, require developing novel manufacturing methods to ensure high-quality device production. Researchers are currently working on more sustainable manufacturing methods.”
In addition, GaN semiconductors have the potential for material defects and reliability issues including dislocations and point defects which can affect the performance and reliability of the device. Researchers and manufacturers are currently working to improve crystal growth techniques to minimize these defects and enhance the overall quality of GaN materials.
GaN, with its capability to operate more efficiently at higher voltages, temperatures, and switching frequencies than existing silicon devices, is being adopted in both defense and commercial applications. It’s helping to improve system performance in applications that struggle to meet next-generation systems’ requirements.
But, just as GaN is replacing silicon as the go-to technology of choice, what’s going to replace GaN, and just how much longer until that happens?
A Century Of Silicon
Semiconductors are materials that have an electrical conductivity value falling between that of a conductor (such as copper) and an insulator (such as glass) and were first recognized by Thomas Johann Seebeck in 1821. Twenty-two years later, Michael Faraday reported that the resistance of specimens of silver sulfide decreases when they are heated, something that runs contrary to the behavior of metallic substances.
Other milestones occurred during the mid-to-late 1800s, including Alexandre Edmond Becquerel’s observation of a voltage between a solid and a liquid electrolyte, when struck by light, the photovoltaic effect in 1839. This was followed by Willoughby Smith’s observation that selenium resistors exhibit decreasing resistance when light falls on them in 1873, then by Karl Ferdinand Braun’s observation of conduction and rectification in metallic sulfides a year later.
Then, in 1901, the first semiconductor device, called “cat whiskers,” was patented for use in early radio receivers. Twenty-five years later, Field Effect Semiconductor Device Concepts were patented and, in 1947, John Bardeen and Walter Brattain of Bell Laboratories invented the point-contact transistor. A year later, William Shockley invented the junction transistor, heralding the arrival of the transistor era. For their contribution to semiconductor research and the development of the transistor, Shockley, Bardeen, and Brattain were jointly awarded the Nobel Prize in Physics in 1956.
Finally, in 1954, the first silicon transistor was produced, ushering in the age of silicon electronics and microelectronics. Silicon is, according to Hitachi, found in soil, rocks, water, trees, and plants and is the second most abundant element on Earth after Oxygen.
“In nature, however, silicon is found in the form of compounds with Oxygen, Aluminum, and Magnesium,” writes Hitachi. “As a result, the silicon element must be extracted from the compound and purified. Silicon used in semiconductors such as integrated circuits (IC) requires a single crystal structure of ultra-high purity “99.999999999%” … and is refined using various processes after extraction.”
Silicon enjoyed a nice run as the element of choice for the semiconductor industry and is still, today, commonly used. But in the past thirty years or so, GaN has been chipping away at silicon’s dominance.
The Rise Of GaN
“The existence of gallium (Ga) was first predicted by Dmitri Mendeleev in 1871, and a few years later, in 1875, it was discovered by Paul-Émile Lecoq de Boisbaudran in Paris,” writes EDN. Half a decade later, the first polycrystalline GaN material was synthesized by flowing ammonia (NH3) over liquid gallium.
George Herbert Jones Laboratory synthesized GaN material in 1932 by “reacting gallium metal and ammonia at a very high temperature of 900⁰C to 1,000⁰C,” EDN writes. “And 37 years later, in 1969, H.P. Maruska and J.J. Tietjen reported on the growth of single-crystal film of GaN. They used a hydride vapor phase epitaxy (HVPE) process for the first time to deposit GaN on a sapphire substrate.”
In 1972, J.I. Pankove, E.A. Miller, and J.E. Berkeyheiser developed a blue light detector using GaN. Although it produced blue luminescence at room temperature, its power efficiency was poor. The emergence of growth technologies like metal-organic chemical vapor deposition (MOCVD) and molecular beam epitaxy (MBE) during the 1980s renewed efforts toward the commercial use of GaN. Today, both MBE and MOCVD technologies are used in fabricating GaN devices, depending on the application.
GaN’s coming out party occurred in 1991 when a GaN-based method to produce high-brightness blue LEDs was patented. Two years later, the researchers – Shuji Nakamura, Isamu Akasaki, and Hiroshi Amano – demonstrated these high-brightness blue LEDs, which doped GaN with excess amounts of magnesium. Like Shockley, Bardeen, and Brattain before them, Nakamura, Akasaki, and Amano won a Nobel Prize in Physics in 2014.
“In 2004, Eudyna Device Inc. in Japan introduced GaN-based high electron mobility transistors (HEMT) as depletion mode transistors for RF applications,” writes EDN. “The HEMT structure was based on the phenomenon first described by Takashi Mimura and his team in 1975. Next, in 1994, M. A. Khan and his colleagues demonstrated unusually high electron mobility described as a two-dimensional electron gas (2DEG) near the interface between an AlGaN and GaN heterostructure interface.”
Khan's group demonstrated the first-ever GaN/AlxGaN heterostructure grown using MOCVD. Compared to bulk GaN of the same thickness, this heterostructure showed 12 times higher electron mobility at room temperature. These findings highlight the potential of GaN in power electronics. Eudyna monetized this work to produce benchmark power gain in the multi-gigahertz frequency range.
Nitronex Corporation introduced the first depletion-mode RF HEMT transistor made with GaN grown on silicon wafers a year later, marking the point in time at which GaN RF transistors began making inroads in the RF industry. Limited by device cost, work began on GaN FETs “by growing a thin layer of GaN on the aluminum nitride (AIN) layer of a standard silicon wafer using the MOCVD technique, in which the material layers are created through highly controlled chemical reactions among gasses released into a chamber. The AIN layer acts as a buffer between the substrate and GaN.”
Enhancement-mode GaN on silicon wafers designed specifically as power MOSFET replacements were introduced by Efficient Power Conversion in 2009, opening the door for higher-volume/lower-cost GaN production using standard silicon manufacturing technology and facilities. This development led industry heavyweights Fujitsu, MicroGaN, Panasonic, and Texas Instruments to begin developing their versions of GaN devices.
Enhancement-mode GaN transistors were created as a substitute for power MOSFETs in situations where high-speed switching or power conversion efficiency is vital. Following their introduction, researchers started working on GaN power ICs, which would combine a GaN FET, GaN-based drive circuitry, and circuit protection into one surface-mount device.
A gate-drive loop with essentially zero impedance was achieved through this integration, which significantly improved efficiency by eliminating FET’s turn-off losses. The development of low-voltage GaN power ICs started at the Hong Kong University of Science and Technology, and the first devices were showcased in 2015. Later in 2016, HRL Laboratories in Malibu, CA demonstrated a GaN power IC, which enabled the realization of all the benefits of GaN electronics at a low cost.
“Most of the challenges associated with the early implementations of GaN devices, such as reliability, supplier diversity, and production capacity have been solved, writes UnWired. “However, the cost of GaN devices has become an oft-cited impediment when looking to understand the future of GaN as a replacement for SiC-based products.”
Even with that impediment, the global GaN market – estimated at $750 million in 2022 – is forecast to surpass $5 billion by 2032, according to Fact.MR. “In recent times, the introduction of 4G LTE and 4G Volte uplifted the market scenario of GaN RF power devices,” adds Fact.MR. “Moreover, numerous technical advancements incurred in this domain empowered the top five GaN RF device manufacturers to capture around 16% of the overall GaN RF device market.
“Attributed to future projections for GaN RF components, they are projected to grow at a stupendous rate of 21.3% during the next decade. Exceptional growth is anticipated for the aforementioned devices due to product expansions and technical improvements associated with the telecommunication industry. The expected shift from 4G to 5G network will boost the demand for GaN RF devices in telecommunications to grow at a CAGR of 24.8% during the forecast period.”
Long Live The King
Which brings us back to where we started: Is GaN still hot? Much like GaN is slowly replacing silicon, something out there is lurking at a replacement for GaN.
One scenario is that silicon survives the GaN challenge and remains the element of choice due to it being less expensive, as well as its excellent electrical conductivity and thermal stability. Germanium has been mentioned as well as it is not as rare as gallium, but it is still a relatively expensive material.
“Graphene is a 2D material with an enormous amount of flexibility in terms of how it can be formed,” writes FindLight. “The potential applications of graphene are vast, ranging from medicine to solar energy, to sensing technology. The most pertinent use is certainly in electronics.
“Graphene is the most conductive material that material researchers know of. Microchips that use graphene can sustain many more transistors than commonly used materials like silicon. This alone will make electronics more efficient. The real benefit that graphene can provide is in the elimination of carbon created in silicon refinement.”
Perovskites are emerging as a potential alternative to silicon in the field of solar energy and photovoltaics. Unlike the process of laser refinement that is used to create monocrystalline or polycrystalline silicon, perovskites are much more tolerant to imperfections. This means that perovskites do not require the same high standard of purity as silicon to perform their functions effectively.
“Perovskites’ key limitation is that it is not stable enough for mass production in commercial quantities,” FindLight writes. “Many variables can cause damage or imperfections that must be smoothed out before perovskite is deployed in masse for solar energy cells.”
Neither silicon nor GaN will be replaced any time soon. But looking back on two hundred years of semiconductor history, it’s obvious that change is constant, and technological revolutions are a common occurrence. As to whether GaN will mirror silicon’s 100-year run as an element of choice is going to happen, only time will tell.