What's Better – TWTAs Or SSPAs?
By Mike Lee, dB Control
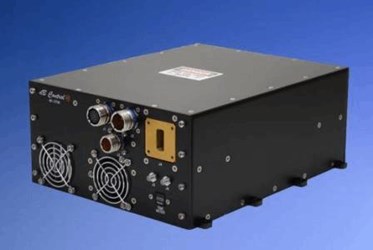
By Mike Lee, dB Control
The short answer is: it depends on the application.
First off, what is an amplifier? The amplifier is an electronic device that increases or “amplifies” the voltage, current, or power of a signal. Amplifiers in the context of this paper are used in wireless communication, broadcasting, airborne datalink, RADAR, electronic warfare (EW), and electronic countermeasure (ECM) applications. Traveling wave tube amplifiers (TWTAs) and solid state power amplifiers (SSPAs) are the subjects of the comparison in this paper.
access the Guest Column!
Log In
Get unlimited access to:
Trend and Thought Leadership Articles
Case Studies & White Papers
Extensive Product Database
Members-Only Premium Content
Welcome Back! Please Log In to Continue.
X
Enter your credentials below to log in. Not yet a member of RF Globalnet? Subscribe today.
Subscribe to RF Globalnet
X
Subscribe to RF Globalnet