Maser: Laser's Predecessor Loses Its Refrigerator
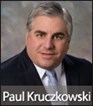
By Paul Kruczkowski, Editor
Researchers at the UK’s National Physical Laboratory (NPL) and Imperial College London have demonstrated the first room-temperature, zero-field microwave amplification by stimulated emission of radiation, or maser. This groundbreaking work opens the door for the practical application of masers as microwave low noise amplifiers (LNAs).
Masers have been around since the early 1950s, several years before the laser was invented. However, maser use has been limited to special LNA applications, like NASA’s deep space network (DSN) dish antennas that send and receive messages to our space exploration vehicles like the Mars Curiosity Rover. This is due to the fact that traditional masers were operated in a vacuum, within a strong magnetic field, and at cryogenic temperatures — all of which required bulky and expensive equipment and regular maintenance.
How The New Maser Works
The new device tested at NPL replaces the ruby traditionally used to amplify the microwaves with a new organic crystal material called p-terphenyl doped to 0.01% with pentacene. The device is optically pumped with a pulsed yellow dye laser (~585 nm) that is currently used in the medical field to treat skin lesions. The p-terphenyl crystal rests on a sapphire dielectric support within a silver-plated, high-Q cavity tuned to resonate at 1.45 GHz.
The pulsed laser is focused on the p-terphenyl crystal to pump or excite the pentacene molecules to their first-excited single state. The optically excited pentacene molecules undergo inter-system crossing into their triplet ground state. This process is spin selective, and the highest of three spin levels is favored, creating a population inversion between the highest and the lowest spin levels. This triplet mechanism and population inversion provides the conditions for masing, as molecules transition from the highest to lowest spin level, and does so without the aid of an applied magnetic field.
Fields are generated within the resonant cavity, and a -10 dBm signal at 1.45 GHz is coupled from the magnetic field using inductive coupling loop. The intrinsic noise temperature of the room-temperature maser is only a few hundred milli-Kelvins, with the caveat that it was only pulsed for duration of a few hundred microseconds for this demonstration.
Future Prospects For Maser Technology
Building a device that finally demonstrates masing without the need for extreme cooling, vacuum chambers, or external magnetic fields is a major achievement that paves the way for the development of new masers unencumbered by the large and costly support systems of current maser technology. When I spoke to NPL’s Dr. Mark Oxborrow about the team’s next steps for the technology, he told me that they were focused on enhancing the first device through continuous operation of the pump laser, improved focus of the pump laser, and enhanced quality of the crystal. He added that they were formulating ideas for future masers that are smaller, target other frequencies, and improve power consumption.
While maser technology’s inherent limited gain bandwidth product does limit it to narrow band applications (less than 100 MHz), its high gain and ultra-low thermal noise temperature make it ideal for ultra-sensitive detection of extremely weak signals. The NPL/Imperial College London breakthrough should help expand the use of masers for more accurate space telescopes, satellites, and deep-space communications. In addition, smaller, less-expensive masers may someday improve sensors in healthcare with higher definition microwave scanners, and in security with better chemical and bomb detection. It will be exciting to watch the technology develop, and only time will tell if the maser can find as many applications as its younger sibling, the laser, has since its inception.
How do you think masers will be applied in the future? Will they ever achieve “laser” status and be a commonly used device?