Injector Gun, Cryomodule Delivered For SLAC's X-Ray Laser Upgrade
By Jof Enriquez,
Follow me on Twitter @jofenriq
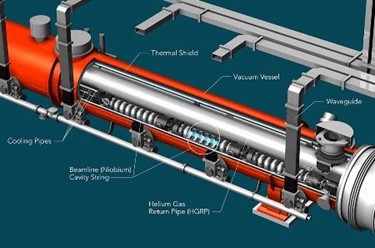
The injector gun and first cryomodule for Linac Coherent Light Source II (LCLS-II) — one of the most advanced superconducting laser projects in the world and the only one in the U.S. — have arrived at the U.S. Department of Energy’s (DOE) SLAC National Accelerator Laboratory in Menlo Park, California. The components are part of a major upgrade of SLAC's X-ray free-electron laser that scientists will use to further understand the fundamentals of atomic and quantum physics.
Designed and built by Berkeley Lab, the injector gun features a copper-based radio-frequency (RF) accelerating cavity which shoots electrons excited by an ultraviolet (UV) frequency laser into the superconducting particle accelerator. A steady stream of these electrons will then be used to produce ultrabright X-ray laser pulses at an unprecedented rate. The injector source was delivered at SLAC on Jan. 22, 2018.
"The performance of the injector gun is a critical component that drives the overall operation of our X-ray laser facility, so we greatly look forward to seeing this system in operation at SLAC," said Mike Dunne, LCLS director at SLAC. "The leap from 120 pulses per second to 1 million per second will be truly transformational for our science program."
The bulk of the superconducting particle accelerator will be made up of 37 cryomodules — 40-foot-long-by-4-foot-wide sections of superconducting metal cylinders with cooling components. The first of these was delivered Jan. 19, 2018, by Fermilab, which will provide 22 of the cryomodules. DOE’s Thomas Jefferson National Accelerator Facility in Virginia will build the rest.
Fermilab says the quality factor achieved in the cryomodule doubles that of state-of-the-art superconducting RF cryomodules. The higher the quality factor, the lower the cryogenic load, and the more efficiently the cavity imparts energy to the particle beam.
To keep the accelerating cavities inside the superconducting metal below 2 Kelvin (minus 456 degrees Fahrenheit), Fermilab's engineers designed the cryomodule to be fed ultracold liquid helium, flowing through pipes from an aboveground cooling plant. Ultracooling boosts electrons to high energies with minimal energy loss as they travel through the cavities.
"The difference between room temperature and a few Kelvin creates a problem, one that manifests as vibrations in the cryomodule," said Genfa Wu, a Fermilab scientist working on LCLS-II. “And vibrations are bad for linear accelerator operation."
The lab's scientists substantially reduced vibration levels — to one-tenth of what they were originally — by adjusting the flow path of the liquid helium, among other changes.
Inside the cryomodules, three layers of cooling equipment lower the temperature to nearly absolute zero. These surround eight superconducting niobium cavities bolted together in a string. Each cavity is filled with electric fields that accelerate electrons to nearly the speed of light.
“It’s safe to say that this is the most advanced machine of its type,” said Elvin Harms, a Fermilab accelerator physicist working on the project. “This upgrade will boost the power of LCLS, allowing it to deliver X-ray laser beams that are 10,000 times brighter than it can give us right now.”
As a next-generation X-ray laser, LCLS-II at SLAC will help scientists meet ambitious research goals: examine the details of complex materials with unparalleled resolution, reveal rare and transient chemical events, study how biological molecules perform life’s functions, and peer into the strange world of quantum mechanics by directly measuring the internal motions of individual atoms and molecules.