Future Trends In Radar And Antenna Technology: A Comprehensive Overview

By John Oncea, Editor
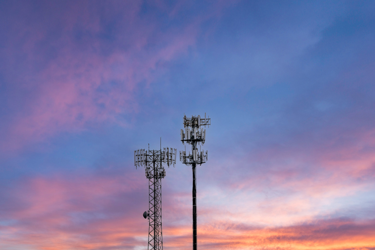
Radar relies on antennas to transmit and receive signals, enabling various applications from traffic control to space surveillance. Future advancements focus on digital and cognitive systems for enhanced performance.
Radars and antennas go hand in hand – without an antenna, a radar is useless. And yes, some radar designs use multiple stationary antenna arrays instead of a single rotating antenna, but they still require antennas to transmit and receive the signals.
The antenna’s performance is based on its ability to detect the exact direction of an object. The antenna’s size determines the beam angle, with longer antennas producing narrower beams that can better distinguish between nearby objects.
Radar uses antennas to both transmit and receive radio waves. First, the radar antenna transmits pulsed electromagnetic waves into the environment, usually in the microwave range. These waves travel outward and can reflect off objects, creating echoes. The same antenna then receives the reflected echoes from the objects. By analyzing the time it takes for the echoes to return, the radar can determine the distance to the detected objects.
The directionality and focusing of the antenna’s radiation pattern allow the radar to determine the direction and angle of the detected objects. The more antenna elements and the longer the antenna, the more precise the directional information.
All of this allows radars to be used in many ways, including:
- Air and terrestrial traffic control
- Radar astronomy
- Air-defense systems
- Anti-missile systems
- Marine radars
- Aircraft anti-collision systems
- Ocean surveillance systems
- Outer space surveillance and rendezvous systems
The future of radar technology is moving toward digital, software-defined, and cognitive systems. These systems will use the latest semiconductor, signal processing, and antenna technologies to provide more advanced features across various applications. Let’s look at some of the advancements being made in radar and antenna technology.
Stealthy, Quantum, Space-Based Radar
The development of radar technology continues to advance on multiple fronts, driven by both military and civilian applications. Smaller, more efficient, and scalable radar components are enabling more compact and flexible radar systems.
Increased demand for FPGAs, processors, and high-speed ADCs/DACs, according to Pasternack, is driving the need for associated components like high-speed RAM, solid-state drives, and embedded computers. In addition, radar signal chains are becoming more complex, combining advanced transmit and receive capabilities to handle high power levels, wide bandwidths, and complex modulations.
Finally, new test and measurement equipment is required to keep up with the increased agility and programmability of modern radar systems, including real-time spectrum analyzers, vector network analyzers, and advanced signal generators.
Meeting these demands is creating radar systems that are more sophisticated to detect stealthy targets. Researchers are focusing on developing radar systems with enhanced sensitivity, wider bandwidths, and advanced signal processing algorithms to detect and track low-observable targets more effectively.
Progress is being made on quantum radar as well and it has the potential to revolutionize radar technology. Quantum radar promises improved sensitivity and resolution, as well as enhanced security through quantum encryption techniques. Research in this field is ongoing, focusing on overcoming technical challenges and scaling up quantum radar systems.
Radar isn’t only getting more sophisticated, it is ramping up to provide global coverage and persistent monitoring capabilities, making them valuable for various applications including surveillance, environmental monitoring, and disaster management. Advances in satellite technology, radar miniaturization, and launch capabilities are driving the development of space-based radar systems with improved resolution, revisit rates, and coverage.
These are just a few of the many technological advances being made with radars. Others include:
- Digitalization and Software-Defined Radars: Radars are transitioning from legacy analog systems to new digital, software-defined architectures, writes Leonardo. This allows for more flexible, resilient, and higher-performance radar systems that can perform multiple missions with a single platform.
- Passive and Multistatic Radar: Radars that utilize existing electromagnetic transmissions, such as FM radio or digital TV signals, rather than generating their own signals are being developed. These "passive" radars offer a stealthy, low-power alternative for applications like air traffic monitoring.
- Direct RF Sampling and Simplified RF Front Ends: Advances in high-speed analog-to-digital converters (ADCs) enable direct RF sampling, eliminating the need for upconversion/downconversion stages, Emerson writes. This simplifies the RF front-end architecture of active electronically scanned array (AESA) radars, reducing cost and complexity.
- Cognitive and Machine Learning-Enabled Radars: The increased computational power of modern FPGAs allows the integration of machine learning techniques into radar systems. This enables cognitive radars that can automatically adapt their operating parameters and provide advanced capabilities like automatic target recognition.
- Solid-State and Frequency-Stable Transmitters: Replacing traditional magnetron transmitters with solid-state transmitters provides instant power-up, higher reliability, lower power consumption, and frequency stability – improving detection capabilities.
Advancements are being made in the areas of more compact, efficient, and integrated radar components; increased complexity and programmability of radar signal chains; and the use of higher frequency X-band radar with phased array antennas to achieve enhanced resolution, sensitivity, and imaging capabilities. Overall, the future of radar technology is likely to be characterized by increased integration with other sensor modalities, such as electro-optical and infrared sensors, as well as advances in data fusion, networking, and autonomy to support collaborative sensing and decision-making in complex operational scenarios.
Smaller, Smarter Antennas
Antennas come in all shapes and sizes, from small ones that can be found on cell phones to large ones that are used to transmit radio waves over long distances. They are an essential part of modern communication systems and their development promises to be dynamic.
They also need to be modified to meet new usage needs, including miniaturization. As technology becomes smaller and more integrated into everyday objects, there's a growing demand for smaller antennas that can fit into compact devices like wearables, IoT sensors, and even within the structure of clothing.
Antenna miniaturization is achieved by combining metamaterial-inspired designs, reactive impedance surfaces, novel geometries, high dielectric materials, and structural modifications. These techniques allow for significant reductions in antenna size while maintaining acceptable performance.
- Metamaterial-inspired designs: Metamaterials with negative permittivity or permeability can miniaturize antennas, writes IntechOpen. For example, complementary split-ring resonators (CSRRs) etched in the ground plane can reduce the size of patch antennas by over 50%.
- Reactive impedance surfaces: IEEE writes that reactive impedance surfaces (RIS) can be used as antenna substrates to miniaturize the antenna size while enhancing bandwidth and efficiency. A RIS acts as a tunable surface between a perfect electric conductor (PEC) and a perfect magnetic conductor (PMC), allowing optimal miniaturization.
- Novel geometries: Fractal, meandered, and other novel antenna geometries can reduce the physical size of antennas without significantly impacting performance.
- High dielectric materials: Using high dielectric constant materials as the antenna substrate can reduce the physical size, though this may come at the cost of reduced bandwidth and efficiency.
- Slots and slits: Introducing slots, slits, and other structural modifications to the antenna can miniaturize the design without major performance degradation.
Smart antennas are another developing technology resulting in antennas that can dynamically adjust their radiation pattern or other characteristics to optimize performance, improve signal quality, and mitigate interference. Also known as adaptive array antennas, digital antenna arrays, multiple antennas, or MIMO (Multiple-input multiple-output communications), smart antennas are antenna arrays combined with smart signal processing algorithms for identifying spatial signal signatures such as the direction of arrival (DOA) of the signal.
Smart antennas, according to JEM Engineering, can increase the useful signal while reducing interference, improving the signal-to-interference ratio (SIR). They also have directionality that allows them to focus energy toward the intended user, rather than wasting it in unnecessary directions.
They also can locate users which enables features like geo-location services and offer enhanced security as intruders must be positioned in the same direction as the intended user to successfully tap into the connection.
There are two main types of smart antennas, a switched-beam system that chooses from a set of predefined beam patterns to enhance the received signal and an adaptive array that can dynamically steer the beam to the direction of interest while nulling interfering signals. Overall, smart antennas leverage advanced signal processing to optimize antenna performance, increase efficiency, and enable new capabilities like improved coverage, interference reduction, and user tracking.
Other advances occurring in the antenna market are:
- Multi-band and Wideband Antennas: With the proliferation of wireless communication standards, there's a need for antennas that can operate across multiple frequency bands or have wideband capabilities to support diverse communication protocols efficiently.
- Beamforming and Phased Arrays: These technologies enable antennas to focus their signal in specific directions, enhancing communication range, improving signal quality, and reducing interference.
- 5G and Beyond: The rollout of 5G networks presents new challenges and opportunities for antenna development, including higher frequency bands, massive MIMO, and beamforming techniques to support ultra-fast data rates and low-latency communication.
- Energy Harvesting: Antennas can be designed not only for communication but also for harvesting ambient RF energy, which can be used to power low-power electronic devices or sensors in IoT applications.
- Higher Antenna Counts: Radar systems are using more co-located antennas to improve their capabilities. A higher number of antennas can result in higher resolution, higher gain, longer range, wider scanning field of view, and MIMO functionality for tracking multiple objects.